Translational Perioperative and Pain Medicine (ISSN: 2330-4871)
ARTICLE DOI: 10.31480/2330-4871/173
Brief Report | Volume 10 | Issue 2 Open Access
Interaction of Fentanyl with Alpha 2B Adrenergic Receptor
Renyu Liu, MD, PhD*, John Grothusen, PhD, Thomas T. Joseph, MD, PhD and Andrew McKinstry-Wu, MD
Department of Anesthesiology and Critical Care, Perelman School of Medicine, University of Pennsylvania, USA
Renyu Liu, MD, PhD, Professor, Departments of Anesthesiology and Critical Care, and Neurology, Perelman School of Medicine at the University of Pennsylvania, 336 John Morgan Building, 3620 Hamilton Walk, Philadelphia, PA 19104, USA, E-mail: RenYu.Liu@pennmedicine.upenn.eduEditor: Yuan-Xiang Tao, Ph.D., M.D., Professor and Vice Chair, Director, Center for Pain Medicine Research, Department of Anesthesiology, Editor in Chief, The Translational Perioperative and Pain Medicine, Rutgers, The State University of New Jersey, New Jersey Medical School, 185 S. Orange Ave., MSB, E-661, Newark, NJ 07103, USA, Tel: 973-972-9812, E-mail: yt211@njms.rutgers.edu
Received: June 06, 2023 | Accepted: June 15, 2023 | Published: June 20, 2023
Citation: Liu R, Grothusen J, Joseph TT, Wu AM. Interaction of Fentanyl with Alpha 2B Adrenergic Receptor. Transl Perioper Pain Med 2023; 10(2):522-525
Summary
The combined use of fentanyl or other synthetic opioids with xylazine, a non-selective α2 adrenergic agonist approved for use as a veterinary sedative, is increasingly associated with drug overdose deaths. This brief report decribes the molecular interaction between fentanyl and the α2B adrenergic receptor. We tested potential molecular interaction with all of the α-adrenergic receptors using a primary screening method followed by a secondary binding assay to determine the binding affinity. The binding site information was further explored using docking calculations. The results clearly indicated that fentanyl binds to the α2B adrenergic receptor with a Ki of 950 nM and it binds to the intrinsic binding site of the receptor as predicted by the docking experiment. This finding is important because it indicates the cross interaction between fentanyl and xylazine. Further studies are needed to explore the downsteam pharmacological pathways to fully understand the clinical pharmacological effects of co-administration of fentnayl and xylazine.
Introduction
The combined use of fentanyl or other synthetic opioids with xylazine, a non-selective α2 adrenergic agonist approved for use as a veterinary sedative [1], is increasingly associated with drug overdose deaths and reports of necrosis at injection sites. Fentanyl acts primarily through direct binding of the µ opioid receptor, while xylazine nonselectively binds to all four α2 adrenergic receptor subtypes and is a weak agonist at three (A, B, and C) [2]. Fentanyl displaces radiolabeled nonspecific α1 antagonists on α1A, α1B, and α1D receptors and attenuates adrenergic-mediated contraction in aortic and pulmonary arterial smooth muscle [3-5], but the extent of its interaction with α2 adrenergic receptors is not well known. In isolation its adrenergic effects are weak. Yet the profound effects, including death with combined administration of fentanyl and xylazine, invites the hypothesis that there are synergistic interactions among α2 adrenergic agonists, synthetic opioids, and their receptor targets. Such speculation seems particularly warranted given that the receptor targets of both drugs are rhodopsin-like G-coupled protein receptors. As a first step toward testing this hypothesis, we screened for interactions between fentanyl and adrenergic receptors. We further characterized a significant interaction between fentanyl and the adrenergic α2B receptor, with which fentanyl has not previously been reported to interact.
Methods
Radioligand binding assays and affinity determination
Fentanyl was purchased from Millipore-Sigma (> 98% purity). We screened for potential interactions of fentanyl with adrenergic receptors- α1A, α1B, α1D, α2A, α2B, α2C, β1, β2, β3-using a radioligand inhibition screen as previously described [6]. Where potential interactions were found, we performed a secondary binding assay to determine affinity, also as described previously [6]. In brief, radioactive rauwolscine (5 nM) in aliquots of 50 µl were added to wells of a 96-well plate, each of which contained 25 µl aliquots of 10 μM fentanyl and 50 µl of a crude membrane fraction of cells expressing the adrenergic receptors was applied to each well. Reaction incubation, harvesting, and radioactivity measurement procedures from the primary assay were repeated four times to ensure reproducibility. A positive control reference ligand was used for each receptor type. For example, in the case of the α2B adrenergic receptor, the known α2 antagonist yohimbine served as a positive control. Affinity is recorded as the inhibition constant Ki or pKi (−logKi), indicating the concentration of fentanyl required to achieve half maximal inhibition.
Binding site determination and affinity prediction
To locate and visualize the putative binding site, we performed docking simulations using Docking-Server (https://www.dockingserver.com/, Virtua Drug Ltd, Budapest, Hungary) as described previously [6]. We used a published cryo-EM structure of the α2B adrenergic receptor (Protein Data Bank: 6K41) for all docking calculations [7]. Briefly, the protein was cleaned using the docking server, and a simulation box around the intrinsic binding site was selected. Calculations were performed using the Lamarckian genetic algorithm and the Solis & Wets local search method. Initial parameters for fentanyl were set randomly. Each experiment was derived from 100 runs to reach a maximum of 2500000 energy evaluations. The population size was set to 150. During the simulation, a translational step of 0.2 Å, and quaternion and torsion steps of 5 were applied. The most probable binding orientation and affinity are reported.
Data reporting
The data in Figure 1A are presented as mean ± standard error from four repeats. Efficacy of inhibition is determined using the following model: Y = Bottom + (Top − Bottom)/(1 + 10((LogEC50-X) × HillSlope)), and is the concentration of fentanyl that gives a response half way between Bottom and Top plateaus in the units of the Y axis. The image of the protein graphic was generated with PyMOL molecular graphics systems (PyMOL 2.5, Schrödinger, Inc.).
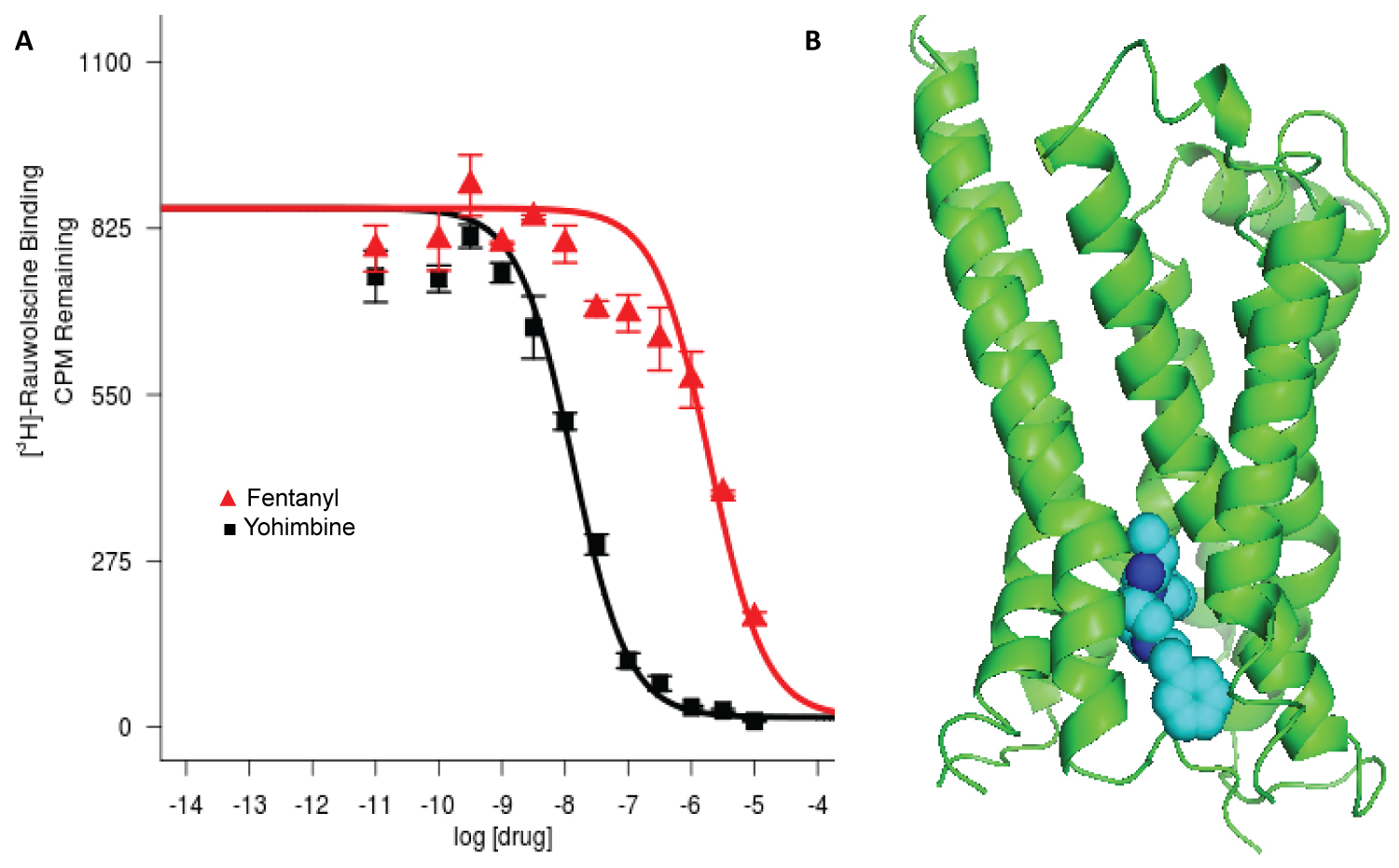
Figure 1: (A) Inhibition of rauwolsine signal (CPM) by increasing concentrations of fentanyl, Ki = 0.95 μM. Yohimbine is used as a positive control. Four repeats were performed as we described previously [6]; (B) Top-ranking docked pose of fentanyl in orthosteric binding pocket of α2B adrenergic receptor.
Results
Affinity of fentanyl to adrenergic receptors
Among the adrenergic receptors tested, only the α2B adrenergic receptor demonstrated significant interaction with fentanyl (Figure 1A). While the initial screening showed some interaction between fentanyl and the α1 receptor, the percentage of inhibition was less than our screening cutoff of 50% displacement. Thus, no further binding assays were performed. Fentanyl diminished the radioactive signal of bound rauwolscine in a dose-dependent manner similar to that of the positive control, yohimbine. The Ki = 0.95 μM indicates a significant interaction with a pharmacologically meaningful affinity.
Identification of binding site
The highest ranked docked pose shows fentanyl binding to the intrinsic ligand binding site in the preformed hydrophobic orthosteric pocket of the α2B adrenergic receptor (Figure 1B). Fentanyl binds in the same pocket in the μ opioid receptor. The interaction mode includes polar, hydrophobic and pi-pi interaction from the top rank docking prediction (Table 1). For the highest ranked pose, the predicted free energy of binding is -8.91 kcal/mol, indicating a predicted dissociation constant KD = 292 nM. Most interactions of fentanyl with amino acid side chains were non-polar in nature.
Table 1: Interaction mechanisms and residues.
Interaction mode |
Interacting residues |
Polar |
THR97 |
Hydrophobic |
LEU89, ALA90, VAL93, CYS96, ILE100, PRO147, LEU166, TRP384, PHE388 |
Pi-pi |
TRP384, PHE387, PHE412, TYR416 |
Other |
ASP92, VAL93, THR97, LEU166, |
Discussion
Fentanyl is a potent opioid with high affinity for the MOR. It is a commonly used medication in the perioperative setting for severe pain management purposes. A full agonist at the MOR, fentanyl’s analgesic potency is much greater than other commonly-used opioids, including morphine and hydromorphone. Along with its potent analgesic effects, fentanyl’s respiratory depressive properties, at least partially mediated by the MOR, is also powerful [8], making it the most lethal opioid in the current opioid crisis [9]. In addition to respiratory depression, fentanyl also reduces cardiovascular function, producing bradycardia and hypotension [10,11]. With its increased usage in the streets, the death toll from fentanyl is skyrocketing [9,12]. This lethality is likely in part because the rapid onset of fentanyl leaves a very short window for intervention and rescue. Co-administration of fentanyl with sedatives or general anesthetics increases the likelihood and severity of respiratory depression with possible respiratory arrest. Xylazine, a sedative approved for veterinary use only, has known side effects that include respiratory depression, bradycardia, and hypotension [13]. It is therefore not surprising that co-administration of xylazine and fentanyl increases the likelihood of respiratory or cardiovascular depression. Unexpectedly, co-administration of the two drugs also appears to cause significantly greater morbidity (respiratory depression, circulatory depression, injection site necrosis) and mortality than expected based on the existing understanding of the pharmacology of fentanyl and xylazine. The molecular mechanisms underlying these amplified effects need to be explored.
In this study, we showed that fentanyl displaces rauwolscine binding at the α2B adrenergic receptor, suggesting that it does, in fact, bind to this receptor. Although the calculated inhibition constant is relatively high, implying a low affinity, this result opens the door to further studies of potential synergistic adrenergic effects of fentanyl and low-potency adrenergic agonists such as xylazine. While our initial screen data did not show significant interaction (no more than 50% inhibition of the reference ligand binding) between fentanyl and the α1 adrenergic receptor, it does not rule out some form of weak interaction between them. It is reported and well-reviewed that it is possible that interaction of fentanyl with the α1 adrenergic receptor contributes to the chest wall rigidity after quick and large doses of fentanyl administration [12]. Further study is needed.
This study shows that fentanyl can bind the α2B adrenergic receptor, but it does not reveal whether it is an agonist or antagonist, nor what signaling pathway it induces or inhibits (i.e. G-protein or β-arrestin). The interaction of fentanyl and xylazine at α adrenergic receptors has not been adequately explored. It is highly possible that fentanyl and xylazine also interact with each other at the MOR, resulting in enhanced opioid-receptor-mediated effects. Further studies exploring these cross-interaction potentials are urgently needed to combat a worsening public health crisis.
Conflict of Interest Statement
None.
Funding Information
The authors acknowldge the support from the following agencies: National Institute of Heath (K08-GM-093115 and 1R01GM111421, PI: RYL; K08 GM139031, PI:TTJ; R35GM14271, PI: AMW); National Institute of Mental Health’s Psychoactive Drug Screening Program, Chapel Hill, North Carolina (NIMH PDSP; Contract# HHSN-271-2008-00025-C).
References
- Friedman J, Montero F, Bourgois P, Wahbi R, Dye D, Goodman-Meza D, Shover C. Xylazine spreads across the US: A growing component of the increasingly synthetic and polysubstance overdose crisis. Drug Alcohol Depend 2022;233:109380.
- Schwartz DD, Clark TP. Affinity of detomidine, medetomidine and xylazine for alpha-2 adrenergic receptor subtypes. J Vet Pharmacol Ther 1998;21:107-111.
- Toda N, Hatano Y. Alpha-adrenergic blocking action of fentanyl on the isolated aorta of the rabbit. Anesthesiology 1977;46:411-416.
- Sohn JT, Ding X, McCune DF, Perez DM, Murray PA. Fentanyl attenuates alpha1B-adrenoceptor-mediated pulmonary artery contraction. Anesthesiology 2005;103:327-334.
- Karasawa F, Iwanov V, Moulds RF. Effects of fentanyl on the rat aorta are mediated by alpha-adrenoceptors rather than by the endothelium. Br J Anaesth 1993;71:877-880.
- Liu R, Huang XP, Yeliseev A, Xi J, Roth BL. Novel molecular targets of dezocine and their clinical implications. Anesthesiology 2014;120:714-723.
- Yuan D, Liu Z, Kaindl J, Maeda S, Zhao J, Sun X, Xu J, Gmeiner P, Wang HW, Kobilka BK. Activation of the alpha(2B) adrenoceptor by the sedative sympatholytic dexmedetomidine. Nat Chem Biol 2020;16:507-512.
- Varshneya NB, Hassanien SH, Holt MC, Stevens DL, Layle NK, Bassman JR, Iula DM, Beardsley PM. Respiratory depressant effects of fentanyl analogs are opioid receptor-mediated. Biochem Pharmacol 2022;195:114805.
- Ciccarone D. The rise of illicit fentanyls, stimulants and the fourth wave of the opioid overdose crisis. Curr Opin Psychiatry 2021;34:344-350.
- Reitan JA, Stengert KB, Wymore ML, Martucci RW. Central vagal control of fentanyl-induced bradycardia during halothane anesthesia. Anesth Analg 1978;57:31-36.
- Lutfy K. Opioid crisis-An emphasis on fentanyl analogs. Brain Sci 2020;10.
- Torralva R, Janowsky A. Noradrenergic Mechanisms in Fentanyl-Mediated Rapid Death Explain Failure of Naloxone in the Opioid Crisis. J Pharmacol Exp Ther 2019;371:453-475.
- Ball NS, Knable BM, Relich TA, Smathers AN, Gionfriddo MR, Nemecek BD, Montepara CA, Guarascio AJ, Covvey JR, Zimmerman DE. Xylazine poisoning: a systematic review. Clin Toxicol (Phila) 2022;60:892-901.