Translational Perioperative and Pain Medicine (ISSN: 2330-4871)
ARTICLE DOI: 10.31480/2330-4871/072
REVIEW ARTICLEOPEN ACCESS
Research Progress of Three-Dimensional (3D) Bio-Printing Technology in Solving Clinical Airway Problems
Jing Lu1,2#, Qian Wu1,2#, Ti-Jun Dai1, Qin Yin1,2, Wei Cheng1,2 , Dun-Yi Q1,2 and Yin-Ming Zeng1,2
1Jiangsu Province Key Laboratory of Anesthesiology and Center for Pain Research and Treatment, Xuzhou Medical University, PR China
2The Affiliated Hospital of Xuzhou Medical University, PR China
#Equal contribution.
Wei Cheng, MD, PhD and Qin Yin, MD, The Affiliated Hospital of Xuzhou Medical University; Jiangsu Province Key Laboratory of Anesthesiology, Xuzhou Medical University, China, E-mail: 53974314@qq.com; 810780794@qq.com
Editor: Renyu Liu, MD, PhD, Associate Professor, Department of Anesthesiology and Critical Care, Perelman School of Medicine at the University of Pennsylvania, 336 John Morgan building, 3620 Hamilton Walk, Philadelphia, PA 19104, Phone: 2157461485, E-mail: liur@uphs.upenn.edu
Received: March 24, 2018 | Accepted: June 15, 2018| Published: June 18, 2018
Citation: Lu J, Wu Q, Ti-Jun D, et al. Research Progress of Three-Dimensional (3D) Bio-Printing Technology in Solving Clinical Airway Problems. Transl Perioper & Pain Med 2018; 5 (3):75-82.
Abstract
The difficult airways caused by congenital or acquired variations are one of the major challenges in clinical anesthesia. The airway tools, airway stents, patches and others nowadays are produced in fixed specification, with complex process, long cycle and poor tissue compatibility, which may increase the risk of infection and difficultly meet the airway variations and airway surgeries. In addition, young anesthesiologists need long time of training before they can independently deal with the difficulty airway. Three-dimensional (3D) bio-printing technology is a promising method in solving clinical airway problems. This article intends to make a summary and prospect of related areas.
Keywords
Three-dimensional, 3D, Anesthesia, Difficulty airway, Airway problems
Introduction
Nowadays 3D printing has been widely used in the medical field and this technology will become a new method for solving clinical airway problems. 3D printing can create individualized airway management tools for patients, which can help anesthesiologists understand the patient's airway specificity before surgery. The mold produced by 3D printing also provides a rare opportunity for young doctors to develop their skills. Of course, this technology still has some ethical and technical conflicts, which requires us to formulate ethical standards and carry out ethical review to guide the correct development of it. In general, 3D printing, as an emerging technology, is subtly changing our lives.
The Role that 3D Bio-Printing Plays in Medical Revolution
The customized, precise and personalized 3D printing technologies will play important roles in the medical revolution during 21st century [1,2]. In recent years, 3D printing is widely used in the areas of artificial scaffolds, tissue and organ transplantation, and medical aids. 3D bio-printing is considered as the most promising new solutions in dealing with the problems of congenital or acquired variations. It is also believed as a new paradigm of tissue engineering and bio-manufacturing in the new century [3-5]. At present, there are many 3D bio-printing researches in Plastic Surgery, Orthopedics, Ear nose throat Branch and other fields, but it is still not popular in the area of airway management [2,6-9].
3D Bio-Printing in Solving the Clinical Airway Problems
The clinical airway problems
Difficult airways causing by congenital or acquired variation need to be solved urgently
In clinical anesthesia, anesthesiologists often encounter variations of anatomy and ventilation resulting from congenital or acquired airway diseases. Due to the limitations of the technologies and experiences, anesthesiologists are difficult to understand the airway variation before surgery, which may threatens patient safety and lead to ventilation failure during anesthesia induction, endotracheal intubation, and intraoperative or postoperative airway management. Therefore, it is an urgent clinical problem to be solved [10].
The fixed types of airway tools are difficult to meet the needs of anatomical variation and personalized ventilation
Currently, most clinical airway management tools in China (tracheal tubes, laryngeal mask, bronchial tubes, etc.) are the dominantly imported products which are designed according to the airway anatomical features of European and American people. These tools are difficult to fully meet the airway status of Chinese people.
Moreover, the fixed types of airway tools producing by fixed molds cannot fully adapt to the individual airway variation, which often leads to unsatisfied ventilation. The choice of ventilation tools mainly depends on the physician's familiarity with the airway tools and their understanding of the airway variations.
Additionally, the production process of ventilation tools traditionally includes producing the mold in advance, melt, injection molding, grinding and other complex processes which need a long production cycle.
The simulation of difficult airway management often neglect the anatomical variations which often lead to a low efficient in training young doctors
As we all known, medical students and young physicians are often confused when they try to understand the anatomical characteristics of airway variations. They have not enough true exercises in clinical patients and also lack of high-fidelity simulation exercises on intuitive models. Therefore, the learning cycle of related airways technologies takes too long to satisfy the educational training of residents and young medical students.
The four levels in dealing with the airway-related clinical issues by 3D bio-printing technology
3D bio-printing technology can be divided into four levels according to the biological properties of materials. Firstly, to produce the medical models and external medical instruments by biological incompatibility and non-degradable materials; Secondly, to produce permanent implants by biocompatible and non-degradable materials; Thirdly, to produce tissue engineering support by biocompatible and degradable materials; Fourthly, to produce manual biological organ or tissues by living cells, proteins, and other extracellular materials [11-16]. These four levels in solving airway-related problems will be introduced respectively as follows.
Firstly, producing airway models and airway instruments for preoperative airway assessment, airway management simulation, doctor-patient communication
3D printing technology in perioperative airway assessment
Neonatology: Before cesarean section, VanKoevering, et al. accurately predicted congenital cleft lip with the help of 3D printing models, and make preparation for potential ventilation difficulties induced by airway variations, so they help the neonate successfully avoid airway problems after the birth [17].
Cardiac surgery: In Cardiothoracic Surgery, it is very common to have patients with congenital airway variation. For such patients, it is very important for doctors to have precise understanding of the adjacent relationships between the variable airway and the cardiovascular structure before surgery. Speggiorin successfully produced a simulation model including the cardiovascular-airway and airway system which help cardiac surgeons familiar with anatomical variations before surgery and make accurate preoperative evaluation, surgical planning and operation preparation [18].
Anesthesiology: Although some clinical randomized controlled trials about 3D printing have been reported in surgical areas, there is not 3D printing related RCT reported in anesthetic fields.
Compared with the traditional preoperative planning, the researchers have produced 1:1 physical airway system model in different facets which help the anesthesiologist observe the anatomical features of a particular area better and develop a accurate individualized airway plan. Furthermore, the anesthesiologists have simulated the tracheal intubation and simulated ventilation for special patients by a 3D print airway model which help them deal with difficult airways and reduce related risks [2,7,19-23]. Wilson, et al. performed 3D reconstruction of the patient's airways and subsequently produced an airway model including the trachea from the larynx level and the main bronchi up to the first bronchial bifurcation by 3D printed technology for those children who need single lung ventilation. Before the operation, the doctor simulated the endotracheal intubation on this model. They made a precise plan: Insert a size 3.5 tube into the right bronchus and a size 4 tube into the left bronchus with the tube's tips at the right positions. They succeeded in carrying out the anesthesia plan for the single lung ventilation during the surgery, and the child received satisfactory ventilation and postoperative recovery [7].
Oral, ENT, Orthopedics, Pulmonary: In 1992, Stoker, et al. [24] firstly introduced 3D printing technology for preoperative simulation in the field of plastic surgery. 3D printing can perfectly shape the mandible implants and repair the bone's defects with the high accuracy, short time, good recovery of oral function and high patient satisfaction according to the postoperative appearance. 3D printing technology have also been applied in maxillofacial surgery, nose and mouth assessment before surgery, simulation of airflow dynamics changes, prediction and evaluation of surgical effects and feasibility in advance [25-28].
At the ClinicalTrials.gov website, we find only one trial about 3D printing in airway field by Morrison (Recruiting, February 8th 2018) [29]. The registration information suggests that some children cannot freely use traditional continuous positive airway pressure mask due to different craniofacial abnormalities. The team firstly re-constructed the 3D model according to patients' anatomical variations, then produced personalized CPAP masks by computer-aided design and 3D printing techniques for solving the poor matching problem of traditional masks.
Preoperative education and simulation training of airway management: The risk of airway surgery is high due to its complex anatomical structures and important structures adjacent to nerves and blood vessels. 3D printing technology can reproduce the anatomical structure of the diseased area in vitro, which is helpful for disease diagnosis, communication between doctors and patients, preoperative planning and designing, surgical simulation, prediction and evaluation of surgical effects. The doctor and the patient can talk about the conditions about the key areas and anatomical features by using the personalized anatomy model, which can help increase their understanding of the disease, to improve the communications between doctors and patients, improve their awareness of the disease, and strengthen the mutual trust between doctors and patients [20]. This could potentially reduce the high medical disputes from poor communications between doctors and patient in China.
At any time during the perioperative period, 3D printing technology can reproduce the important anatomical area of the airway system according to the acquisition. Thereby, it can provide an intuitive, three-dimensional, typical 3D printing physical model for clinical teaching and repeated-practice before surgery. It can also help students and young doctors to have a better understanding of the anatomy and pathogenesis of airway-related diseases, and provide true simulation training before surgery and anesthesiology [30].
The bio-printing of airway systems at Level 2 - customized personalized implants
In difficult airway surgery, the ventilation and surgical effects are highly dependent on the doctor's skills, creativity, and patients' own regenerative potential due to the randomness of airway variation. 3D printing technology provides strong technical support for solving these problems. Before surgery, doctors can convert the image data into various spatial structures directly, make all kinds of materials into implants, and simulate the operation and anesthesia process, which will change the anesthesia and surgical plan and specifications completely [31].
The 3D bio-printing of airway systems at Level 3 - controlled-release drug and tissue engineering stents
The second level of 3D printed airway stents is only for mechanical support and is heterogeneously implanted in nature. The emergence of airway stents and patches which combined the 3D bio-printing techniques with tissue engineering or/and drug sustained-release technology has brought new hope for airway softening disease and airway repair [32-34].
3D printing controlled-release drug stents
Appropriate release of drugs and growth factors is very important for maintaining normal function of tissue and organs. Drug delivery system which determines the rate of the scaffold drugs and the growth factors to arrive at cells, tissues, or organs will ultimately affect the treatment effects. Producing a special internal structure of the stent by 3D printing can releasing drugs continuously and constantly which avoid drug side effects caused by the rapid release. Current technologies have been able to 3D-printed bio-ceramics loaded with Rifampin, Vancomycin and calcium phosphate cement scaffolds loaded with bovine serum albumin (BSA)/vascular endothelial growth factor (VEGF) [35-38].
The clinical dose of Cyclosporin A (CsA) have some systemic side effects (such as nephrotoxicity, hepatotoxicity, nervous system abnormalities, gastrointestinal reactions, and respiratory and circulatory toxicity). Local sustained delivery is an effective way to ensure the clinical effect and reduce the systemic side effects. Song, et al. produced a CsA-loaded 3D drug carrier by 3D printing technology with less side effects for stable and sustained delivery of CsA in vivo [39].
Airway repair: Currently, the use of 3D printing technology for tracheal research focused on tracheostomy repair and so on. Chang, et al. used polycaprolactone (PCL) to print the patch of rabbit tracheal damage. Postoperative tissue compatibility and repair effect experiments confirmed that the 3D printing patch repaired airway function effectively [11]. Tam, et al. printed a tracheobronchial tree for a patient with recurrent polychondritis to guide subsequent tracheostomy or tracheal stent placement [32].
Bioabsorbable tracheal stent model: In a 3-year-old child with "tracheobronchomalacia", an engineer first designed a tracheal stent model suitable for the children with a computer. Then, they printed the absorbable extra-tracheal support bracket with a bioabsorbable material and performed an artificial tracheal implant. The implanted stent worked well and was gradually absorbed by the body. This case foreshadows the tremendous promise of 3D bio-printing technology in personalized airway management [33].
The 3D bio-printing of airway systems at Level 4 - cell, tissue and organ print
Cell printing is an advanced technique for constructing biologically active three-dimensional multicellular systems in vitro. The technology combining rapid prototyping technology with bio-manufacturing technology had printed out the kidney, airway system, blood vessels, skin, bionic tissue and other tissues or organs. This will help solve the problems in traditional tissue engineering and have broad prospects for development in biomedical engineering and applied research [40,41].
Cell printing technology: A variety of cell printing technologies have been developed so far, such as ink jet technology, electrospray technology, air extrusion, laser and voice control technology, spray delivery and so on. Among them, 3D Bioplotter is currently the most commercially available technology model that has been developed to the 4th generation (Biostructural Engineering Bioplotter 3D printer). There are two main technologies commonly used for cell 3D printing: One is based on extrusion or droplet injection molding; the other is based on light pressure or light curing forming. At present, single cell printing is the hot topic in cell 3D printing. There are still many technical bottlenecks in the research of multicellular 3D printing, which still needs more breakthroughs in research and development [42-44].
Organ printing technology: Organ printing is a latest cutting-edge technology assisted by computer design, used a way by layer superimposed, combined cells, cell matrices and other biological materials forming a certain spatial structure. Ultimately, it can build a living certain functional human organ with a three-dimensional and pore structure. The technology will produce the precise construction of complex airway system allowing for automation, customization, efficiency and precision, and is the potential method for in-situ printing. The cell's own replication, the interactions between cells, and the extracellular matrix are naturally occurring self-assembly phenomena [45-47], which is also the biological basis of organ printing.
Although 3D bio-printing has printed a variety of tissues and organs such as the skin, blood vessels, liver, cartilage, heart and so on, no bio-active printed tissue organ has been put into clinical application and no printed bioactive airway stent reported. Due to the complexity of the human airway structure and the function of the organ, future research focuses on printing the living airway cells, the biological scaffold material, and the type of extracellular matrix and the regulation.
Ethical Issues on 3D Biometric Printing
3D bio-printing has made some breakthroughs in artificial tissue. However there is still a long way to accurate human reproduction, the unlimited development of technology has forced us to think about potential ethical issues. There are two main aspects of the ethical issues that are generated by bio-printing the human body parts [48,49]:
First, the reproduction of human tissues and organs through bio-printing can have an impact on the existing social ethics. We can bio-print body tissues and organs similar to the original, but we cannot deny the ethical relationship between the organ and the original organism. These above will bring many uncertainties to the family and intergenerational relationships.
Second, copying people or organs through bio-printing is a challenge to the unique value of humanity, and is contrary to the ethical principles of respect. Bio-printing technology is the product of the culmination of science and technology. When a human being or man's organ is continuously duplicated through such high-tech industries as the industrial products on the pipeline, the position and value of man are also lost. At the initial stage, the technological, bio-printing produces artificial organ can be a waste or have some technical defects "duplicator", which will result in the waste of resources and the contempt of their dignity. The appearance of the duplicator can meet the needs of mankind, such as replacing the laborer with itself and becoming the "storage factory" of transplants, but as Kant said: "Man is a purpose, never to use a man as a means". If the duplicators are regarded as a means to cater to the selfish and desire of the manufacturer, their own dignity and worth cannot be guaranteed.
While we pay close attention to the broad application prospect of 3D bio-printing, we should standardize the development of this technology from the perspective of ethics and security. Faced with the potential ethical risks of bio-printing for the purpose of human reproduction and non-medical treatment, and its impact on the existing patent protection system, we must adopt ethical rules, carry out ethical reviews, improve ethical dialogue mechanisms, complete relevant laws, perfect the industrial policies and other measures to guide the development and application of bio-printing .Only by this way can we achieve the purpose of enhancing human's well-being by technology.
Material Study of 3D Printing Artificial Airway
At present, the medical 3D printing materials commonly used are mainly including poly lactic acid-glycolic acid (PLGA), polycaprolactone (PCL), polyetheretherketone (PEEK), acrylonitrile-butadiene-styrene plastic Ester (PC), etc. [4,50,51]. PCL has a low melting point (about 60 ℃), it can provide malleable mechanical support as the tissue grows, deform as the patient's airway grows over time. So it is particularly suitable for supporting and repairing the deficient pediatrics airway [52]. PEEK has a high melting point, it usually uses laser sintering method for 3D printing. If inorganic materials or other polymers are added, it will effectively improve the PEEK mechanical properties and heat resistance. Food-grade PC can effectively avoid the toxicity of bisphenol A to organisms, it can be used in the field of biomedicine. If it is blended with other aromatic polyesters, it will effectively improve the dimensional stability and mechanical properties of the product [53]. The new material, PLGA, not only has a supportive effect but also gradually hydrolyzes in the body, so it can be used as a sustained-release carrier for making temporary stents [25]. PLA and PCL typically require the use of inorganic materials for reinforcement and toughening, followed by 3D printing by melt deposition. Non-food grade PC and ABS cannot be permitted using in the biomedical and tissue engineering areas, and ABS also has poor dimensional stability.
Regarding of the advantages and disadvantages of the above materials, the future of 3D printing materials research will focus on the following aspects: Increasing the existing materials' mechanical properties and stability by modification; developing more new materials like PLGA; researching bioactive printing materials that have complex pore and structural composite [54,55].
3D Printing Technology Patent Airway Tools and Prospects in China
Han, et al. recently reported that they printed out 3D models of the tracheal morphology in patient with cataract surgery and total laryngectomy. The model helps them devise a pre-anesthesia plan. Previously, our team have reached two patent license related to 3D printing airway in China [12,56]: "A method of making and using bronchial catheters" and "Simulation training model of airway emulation, organ and nerve block punctures". Based on the above theoretical basis and research status, combined with our authorized patents, we imagine using the 3D printing technology to solve clinical airway problems (Figure 1):
Figure 1: The anatomic variation (Tracheobronchial) need an unique ventilation catheter with the help of 3D printing technology.
Left (normal airway): Patients with normal tracheal development underwent lung surgery, double lumen bronchial catheterization diagram;
Right (abnormal airway): The patient's right main bronchus is mutated and there is an additional opening above the right upper lung opening. (A) The place of anatomic variation (Tracheobronchial); (B) The 3D printed endotracheal tube will have an additional side hole just opening at the variant place.
Step I: Imaging
The imaging data (chest radiograph, CT or MRI, et al.) indicates that the patient have variations of anatomy. The data will be divided into suitable slices using different imaging software.
Step II: Reconstruction and ModificationThe slices were then transferred to an imaging workstation for additional 3D and multiplanar reconstructions (using Mimics, and Magics Software, et al.). Subsequently, the slices were joined together to compose a 3D reconstruction image. Then, we modify the side holes and structure in 3D digital ventilation catheter model according to anatomical variation of individual patient. Finally, the 3D digital catheter model is finally adjusted to create a new 3D printing STL files.
Step III: 3D printingThe STL files were directly loaded into the 3D printer to produce unique 3D printing catheter for the special patient.
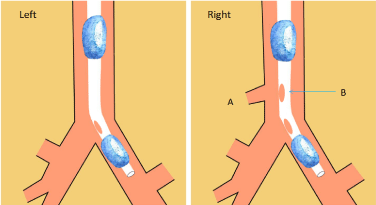
1) Conduct airway routine and imaging assessment for airway deformity patients. Build a three-dimensional database of patients with difficult airway. We aim to build and complete a database of patients with difficult airways by establishing a 3D airway model. To achieve this, a large number of patients with difficult airways will be enrolled in the future study. The databases includes the following contents: General patient status, current medical history, past history, admission diagnosis, planned surgery, specialist examination (the method of assessing difficult airway), imaging data (chest radiograph, CT, fiberoptic bronchoscopy), airway 3D models, anesthesia method, the actual anesthesia process, postoperative visit and so on. The establishment of this database will produce great help for accumulating intubation experience and clinical teaching in difficult airway. It can also provide convenience for the follow-up research.
2) Take patients with difficult airway and airway anatomical variation as the research object. Explore the feasibility of 3D printing technology ventilation tools, and further optimize the airway system 3D printing software and materials technology. The existing 3D bio-printing technology to develop implantable sustained-release airway tools and related materials research can be the preparation for the forthcoming research, which study for biodegradable carrier to treat the personalized airway cancer.
3) Combine animal and clinical test data to conduct in-depth simulation and postoperative actual clinical observation. Clarify airflow dynamics and airway system parameters of the new 3D printing ventilation system. Establish personalized airway management database. Form personalized airway management guidance, and ultimately provide personalized solutions for the accurate medical research in clinical airway management.
4) Create a personalized simulation of the human airway system training model and airway variation 3D printing database, which can be effectively used in clinical teaching simulation exercises and simulation clinical treatment to facilitate and reduce the patients' suffering.
Opportunities and Challenges
It is still in its infancy for 3D printing to solve anesthesia airway problems, there are numerous bottlenecks and challenges. Materials aspects: 3D printed materials are very limited, biomaterials have potential safety issue, low tissue compatibility and high economic costs. Technical aspects: The current 3D printing accuracy, speed and efficiency are not high enough. There are some problems hindering the widespread use of this technology, such as the limited scope of application, the expensive printing equipment and the need for professional training, software design, data processing and multidisciplinary cooperation. Knowledge and Ethics aspects: We should strengthen collaboration between doctors, lawyers and 3D printing professional technicians. In order to train people who are both medical, material science and 3D printing proficiency. More attention should be paid to the ethical issues in hindering the development of 3D printing. There are still many problems and deficiencies in 3D printing technology. However, 3D printing technology has broad application prospects in the field of medical and health in the long run. Just like the computer, half a century ago, no one could have imagined that it would have such huge and far-reaching impacts on human society, in both good and bad way [4,57,58].
Conclusion
Although 3D printing technology has been applied in the anesthetic airways, there are still many technical bottlenecks in this technology. But we believe that in the near future, this emerging technology will be widely used in clinical practice to improve our patient care and outcome.
References
- Devarasetty M, Mazzocchi AR. Applications of Bioengineered 3D Tissue and Tumor Organoids in Drug Development and Precision Medicine: Current and Future. BioDrugs 2018;32:53-68.
- Chao I, Young J, Coles-Black J, et al. The application of three-dimensional printing technology in anaesthesia: a systematic review. Anaesthesia 2017;72:641-650.
- Farooqi KM. Innovations in Preoperative Planning: Insights into Another Dimension Using 3D Printing for Cardiac Disease. J Cardiothorac Vasc Anesth 2017;:.
- Pucci JU, Christophe BR, Sisti JA. Three-dimensional printing: technologies, applications, and limitations in neurosurgery. Biotechnol Adv 2017;35:521-529.
- Butscher A, Bohner M, Hofmann S, et al. Structural and material approaches to bone tissue engineering in powder-based three-dimensional printing. Acta Biomater 2011;7:907-920.
- Gagnon KB, Caine S, Samadi N, et al. Design of a mouse restraint for synchrotron-based computed tomography imaging. J Synchrotron Radiat 2015;22:1297-1300.
- Wilson CA, Arthurs OJ, Black AE, et al. Printed three-dimensional airway model assists planning of single-lung ventilation in a small child. Br J Anaesth 2015;115:616-620.
- Pedersen TH,Gysin J, Wegmann A, et al. A randomised, controlled trial evaluating a low cost, 3D-printed bronchoscopy simulator. Anaesthesia 2017;72:1005-1009.
- Taniguchi D, Matsumoto K, Tsuchiya T, et al. Scaffold-free trachea regeneration by tissue engineering with bio-3D printing. Interact Cardiovasc Thorac Surg 2018;:.
- Mooty RC, Rath P, Self M, et al. Review of tracheo-esophageal fistula associated with endotracheal intubation.J Surg Educ 2007;64:237-240.
- Chang JW, Park SA, Park JK, et al. Tissue-engineered tracheal reconstruction using three-dimensionally printed artificial tracheal graft: preliminary report. Artif Organs 2014;38:E95-E105.
- Wei C, Qin Y, MingYue C, Cheng C, et al. A method of making bronchoducts. China patent A61M16/04(2006.01)I, 201310684433.9(Authorization)
- Whitford WG. A bioink by any other name: terms, concepts and constructions related to 3D bioprinting. Future Sci OA 2016;2:FSO133.
- Yoo S S. 3D-printed biological organs: medical potential and patenting opportunity. Expert OpinTher Pat. 2015, 25(5): 507-511.
- Special Issue: Organs-on-Chips & 3D-Bioprinting Technologies for Personalized Medicine. Stem Cell Rev 2017;13:319-320.
- Chen C, Bang S,Cho Y, et al. Research trends in biomimetic medical materials for tissue engineering: 3D bioprinting, surface modification, nano/micro-technology and clinical aspects in tissue engineering of cartilage and bone. Biomater Res 2016;20:10.
- VanKoevering KK, Morrison RJ, Prabhu SP,et al. Antenatal Three-Dimensional Printing of Aberrant Facial Anatomy. Pediatrics 2015;136:e1382-5.
- Speggiorin S, Durairaj S, Mimic B. Virtual 3D Modeling of Airways in Congenital Heart Defects. Front Pediatr 2016;4:116.
- Fishman JM, Wiles K, Lowdell MW, et al. Airway tissue engineering: an update. Expert Opin Biol Ther 2014;14:1477-1491.
- Parotto M, Jiansen J Q, Abotaiban A, et al. Evaluation of a low-cost, 3D-printed model for bronchoscopy training. Anaesthesiol Intensive Ther. 2017, 49(3): 189-197.
- Goldstein T A, Smith B D, Zeltsman D, et al. Introducing a 3-dimensionally Printed, Tissue-Engineered Graft for Airway Reconstruction: A Pilot Study. Otolaryngol Head Neck Surg. 2015, 153(6): 1001-1006.
- Han B, Liu Y, Zhang X, et al. Three-dimensional printing as an aid to airway evaluation after tracheotomy in a patient with laryngeal carcinoma. BMC Anesthesiol. 2016, 16: 6.
- Hertegard S. Tissue engineering in the larynx and airway. CurrOpinOtolaryngol Head Neck Surg. 2016, 24(6): 469-476.
- Stoker N G, Mankovich N J, Valentino D. Stereolithographic models for surgical planning: preliminary report. J Oral Maxillofac Surg. 1992, 50(5): 466-471.
- Jacobs C A, Lin A Y. A New Classification of Three-Dimensional Printing Technologies: Systematic Review of Three-Dimensional Printing for Patient-Specific CraniomaxillofacialSurgery. PlastReconstr Surg. 2017, 139(5): 1211-1220.
- Pfaff M J, Steinbacher D M. Plastic Surgery Applications Using Three-Dimensional Planning and Computer-Assisted Design and Manufacturing. PlastReconstr Surg. 2016, 137(3): 603e-616e.
- Zhong N, Zhao X. 3D printing for clinical application in otorhinolaryngology. Eur Arch Otorhinolaryngol. 2017, 274(12): 4079-4089.
- Crafts T D, Ellsperman S E, Wannemuehler T J, et al. Three-Dimensional Printing and Its Applications in Otorhinolaryngology-Head and Neck Surgery. Otolaryngol Head Neck Surg. 2017, 156(6): 999-1010.
- Morrison R J. 3D-Printed CPAP Masks for Children With Obstructive Sleep Apnea. ClinicalTrials.gov Identifier: NCT02261857 (Recruiting);2014.
- Byrne T, Yong S A, Steinfort D P. Development and Assessment of a Low-Cost 3D-printed Airway Model for Bronchoscopy Simulation Training. J BronchologyIntervPulmonol. 2016, 23(3): 251-254.
- Hsueh W D, Smith L P. External airway splint to treat tracheomalacia following laryngotracheal reconstruction. Int J Pediatr Otorhinolaryngol. 2017, 94: 68-69.
- Tam M D, Laycock S D, Jayne D, et al. 3-D printouts of the tracheobronchial tree generated from CT images as an aid to management in a case of tracheobronchial chondromalacia caused by relapsing polychondritis. J Radiol Case Rep. 2013, 7(8): 34-43.
- Zopf D A, Hollister S J, Nelson M E, et al. Bioresorbable airway splint created with a three-dimensional printer. N Engl J Med. 2013, 368(21): 2043-2045.
- Ott L M, Zabel T A, Walker N K, et al. Mechanical evaluation of gradient electrospun scaffolds with 3D printed ring reinforcements for tracheal defect repair. Biomed Mater. 2016, 11(2): 25020.
- Genina N, Boetker J P, Colombo S, et al. Anti-tuberculosis drug combination for controlled oral delivery using 3D printed compartmental dosage forms: From drug product design to in vivo testing. J Control Release.2017, 268: 40-48.
- Martinez-Vazquez F J, Cabanas M V, Paris J L, et al. Fabrication of novel Si-doped hydroxyapatite/gelatine scaffolds by rapid prototyping for drug delivery and bone regeneration. ActaBiomater.2015, 15: 200-209.
- Wu G, Wu W G, Zhou J B, et al. Fabrication of Isoniazid/Rifampicin/Poly L-lactic Acid Donut-shaped Implants via Three Dimensional Printing Technique. Zhongguo Yi XueKeXue Yuan XueBao. 2017, 39(6): 743-748.
- Fahimipour F, Rasoulianboroujeni M, Dashtimoghadam E, et al. 3D printed TCP-based scaffold incorporating VEGF-loaded PLGA microspheres for craniofacial tissue engineering. Dent Mater. 2017, 33(11): 1205-1216.
- Song T H, Jang J, Choi Y J, et al. 3D-Printed Drug/Cell Carrier Enabling Effective Release of Cyclosporin A for Xenogeneic Cell-Based Therapy. Cell Transplant. 2015, 24(12): 2513-2525.
- Ahlfeld T, Cidonio G, Kilian D, et al. Development of a clay based bioink for 3D cell printing for skeletal application. Biofabrication. 2017, 9(3): 34103.
- Koo Y, Kim G. New strategy for enhancing in situ cell viability of cell-printing process via piezoelectric transducer-assisted three-dimensional printing. Biofabrication. 2016, 8(2): 25010.
- Park J H, Jang J, Lee J S, et al. Three-Dimensional Printing of Tissue/Organ Analogues Containing Living Cells. Ann Biomed Eng. 2017, 45(1): 180-194.
- Baba M, Matsumoto K, Yamasaki N, et al. Development of a Tailored Thyroid Gland Phantom for Fine-Needle Aspiration Cytology by Three-Dimensional Printing. J Surg Educ. 2017, 74(6): 1039-1046.
- Alkhouri N, Zein N N. Three-dimensional printing and pediatric liver disease. CurrOpinPediatr. 2016, 28(5): 626-630.
- Alyaev Y G, Sirota E S, Bezrukov E A, et al. [Application of 3D soft print models of the kidney for treatment of patients with localized cancer of the kidney (a pilot study). Urologiia. 2017(6): 12-19.
- Nawroth J C, Scudder L L, Halvorson R T, et al. Automated fabrication of photopatterned gelatin hydrogels for organ-on-chips applications. Biofabrication. 2018, 10(2): 25004.
- Suzuki R, Taniguchi N, Uchida F, et al. Transparent model of temporal bone and vestibulocochlear organ made by 3D printing. AnatSci Int. 2018, 93(1): 154-159.
- Cornwall J. The ethics of 3D printing copies of bodies donated for medical education and research: What is there to worry about? Australas Med J. 2016, 9(1): 8-11.
- Neely E L. The Risks of Revolution: Ethical Dilemmas in 3D Printing from a US Perspective. SciEng Ethics. 2016, 22(5): 1285-1297.
- Hoang D, Perrault D, Stevanovic M, et al. Surgical applications of three-dimensional printing: a review of the current literature & how to get started. Ann Transl Med. 2016, 4(23): 456.
- Sears N A, Seshadri D R, Dhavalikar P S, et al. A Review of Three-Dimensional Printing in Tissue Engineering. Tissue Eng Part B Rev. 2016, 22(4): 298-310.
- Yu H S, Park J, Lee H S, et al. Feasibility of Polycaprolactone Scaffolds Fabricated by Three-Dimensional Printing for Tissue Engineering of Tunica Albuginea. World J Mens Health. 2018, 36(1): 66-72.
- Deng X, Zeng Z, Peng B, et al. Mechanical Properties Optimization of Poly-Ether-Ether-Ketone via Fused Deposition Modeling. Materials (Basel).2018, 11(2).
- Zhu W, Pyo S H, Wang P, et al. Three-Dimensional Printing of Bisphenol A-Free Polycarbonates. ACS Appl Mater Interfaces. 2018.
- Schmelzer E, Over P, Gridelli B, et al. Response of Primary Human Bone Marrow Mesenchymal Stromal Cells and Dermal Keratinocytes to Thermal Printer Materials In Vitro. J Med Biol Eng. 2016, 36: 153-167.
- Schmelzer E, Over P, Gridelli B, et al. Response of Primary Human Bone Marrow Mesenchymal Stromal Cells and Dermal Keratinocytes to Thermal Printer Materials In Vitro. J Med Biol Eng. 2016, 36: 153-167.
- Goradia V K. Editorial Commentary: Three-Dimensional Printing and Stem Cells May Be a Game Changer for Recovery After Anterior Cruciate Ligament Reconstruction. Arthroscopy. 2018, 34(1): 180-181.
- Goradia V K. Editorial Commentary: Three-Dimensional Printing and Stem Cells May Be a Game Changer for Recovery After Anterior Cruciate Ligament Reconstruction. Arthroscopy. 2018, 34(1): 180-181.
Table of Contents
- Abstract
- Keywords
- Introduction
- The Role that 3D Bio-Printing Plays in Medical Revolution
- 3D Bio-Printing in Solving the Clinical Airway Problems
- Ethical Issues on 3D Biometric Printing
- Material Study of 3D Printing Artificial Airway
- 3D Printing Technology Patent Airway Tools and Prospects in China
- Opportunities and Challenges
- Conclusion
- Figure 1
- References